Microscope objectives are often interchangeable, but compatibility depends on factors like the thread type, tube length, correction standards, and optical design.
The majority of modern microscopes use the DIN (Deutsche Industrie Norm) or RMS (Royal Microscopical Society) standard for threading, allowing interchangeability across brands that adhere to these specifications. However, for specialized systems or manufacturers, proprietary designs may limit compatibility.
Common standards include metric 25-millimeter and metric 32-millimeter objective threads. Differences in optical qualities, numerical aperture objectives, and specialized coatings for specific applications also limit interchangeability.
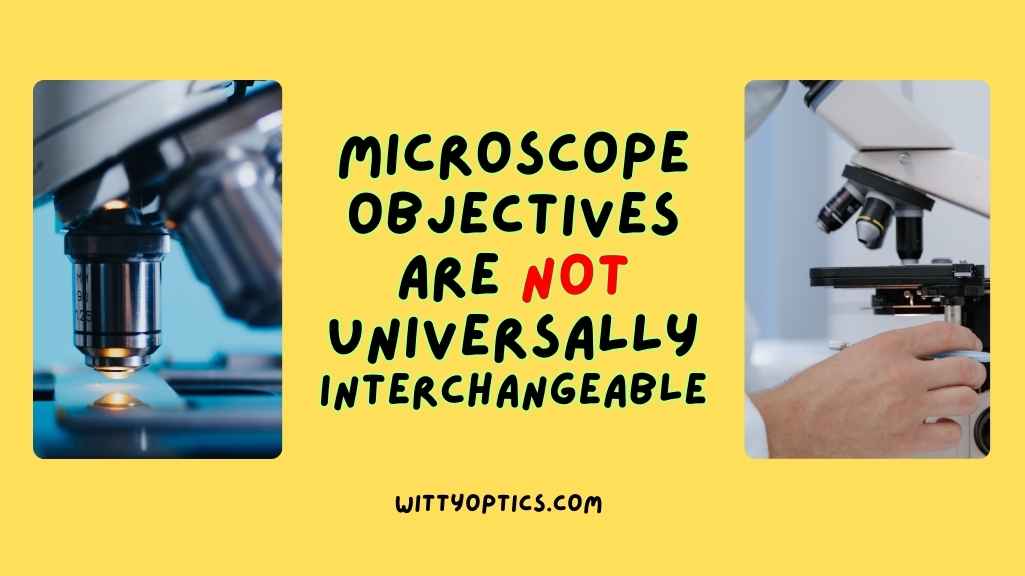
Factor | Details | Common Standards |
---|---|---|
Thread Type | Connection between microscope and lens. | RMS (0.8” diameter, 36 TPI), DIN (M25) |
Tube Length | Determines optical path length. | 160mm (DIN Standard), Infinity Systems |
Correction Type | Corrects spherical/ chromatic aberration. | Achromatic, Semi-apochromatic, Apochromatic |
Magnification | Determines level of detail seen. | 4x, 10x, 40x, 100x (oil immersion) |
Parfocal Distance | Distance from focus plane to mount. | 45mm (DIN Standard), varies otherwise. |
Brand-Specific Designs | Proprietary limits to interchangeability. | Olympus UIS2, Nikon CFI60, Zeiss ICS |
For consistent performance, verify the system specifications and test objectives on your microscope.
5 Key Factors Affecting Interchangeability
The design of microscope objectives plays a significant role in their compatibility with different microscopes. Below are the critical parameters to evaluate:
1. Thread Size and Compatibility
Most microscopes use standardized thread sizes to mount objectives on the nosepiece turret. The most common threads are metric 25-millimeter (RMS thread) and metric 32-millimeter DIN threads. Ensuring the objective’s thread size matches the nosepiece threading is essential for successful mounting.
Different manufacturers may use proprietary thread sizes for specific models, making some objectives incompatible without adapters. It’s recommended to consult the objective manufacturer’s specifications for thread size information.
2. Parfocal Distance
Parfocal distance refers to the distance between the objective’s mounting thread and its focal plane when focused. Microscope objectives commonly have parfocal distances of 45 mm (DIN standard) or 60 mm (JIS standard). If the parfocal distance does not match the mechanical tube design, users may encounter focusing issues.
Parfocal Distance Standard | Typical Value |
---|---|
DIN Objectives | 45 mm |
JIS Objectives | 60 mm |
3. Tube Lens and Infinity Correction
Modern microscopes often use infinity-corrected objectives, where light passes through the objective and continues parallel until it converges at the intermediate image plane with a tube lens. Older models may rely on finite objectives, which focus light directly to the intermediate image plane without a tube lens.
Objectives must match the optical tube design. Using a finite objective in an infinity-corrected microscope can result in image degradation and a loss of optical qualities.
4. Numerical Aperture Values and Imaging Medium
Numerical aperture (NA) defines an objective’s light-gathering power and resolution potential. Differences between immersion objectives and dry objectives can influence their compatibility with certain microscopes. For example:
- Dry objectives operate without immersion liquids.
- Immersion objectives utilize specific media such as water, oil, or glycerin for focusing light waves more effectively.
- Homogeneous immersion ensures refractive indices are matched, minimizing spherical aberration.
Selecting the correct numerical aperture objectives for your application ensures image quality across a wide range of biological or optical microscopy tasks.
5. Color Code Identification and Markings
Microscope manufacturers utilize color-coded markings on objectives for quick identification. For instance:
- Black indicates dark field objectives.
- Blue represents a 40x objective.
Knowing the color codes enables easy identification of objectives when assembling or replacing lenses, reducing compatibility confusion.
Magnification Objective | Color Code |
4x | Red |
10x | Yellow |
40x | Blue |
100x | White |
Optical Corrections in Objectives
Optical aberrations like chromatic aberration and spherical aberration are significant concerns. Microscope objectives incorporate advanced designs to address these issues.
Achromatic Objectives
Achromatic objectives correct chromatic aberration for two colors and spherical aberration for one color, offering basic correction. They are suitable for standard applications requiring economical choices.
Apochromatic Objectives
Apochromatic objectives correct chromatic aberration for three colors and spherical aberration for two. These high-performance objectives deliver sharp, high-contrast images and are suitable for advanced biological applications.
Plan Achromat and Plan Apo Objectives
These objectives provide a flat field of focus across the intermediate image plane, improving imaging media compatibility in cases requiring flatness of field.
Advances in Antireflection Coating Technology
To improve light transmission and minimize reflection losses, objective manufacturers apply antireflection coating technology. Below are the advancements:
- Single-Layer Coatings: Suitable for narrow spectral ranges and basic objectives.
- Multilayer Antireflection Coatings: Offering improved transmittance values and reduced internal reflections across visible wavelengths.
- Magnesium Fluoride Coating: A common material for quarter-wavelength thick antireflection layers.
- Advanced Thin-Layer Coatings: Enhance transmission over a broad range of imaging media and optical qualities.
These coatings significantly improve transmission band performance and are especially useful in high-magnification applications requiring precise light cone control.
Other Design and Mechanical Considerations
Objective Magnification and Barrel Design
Objectives come in various magnifications such as 10x, 40x, and 100x, with barrels often engraved with green or blue color codes for identification. Modern microscope optics incorporate mechanical features like adjustable objectives and correction collars to fine-tune focus for differences between glass or specimens with air.
Magnification Objective | Engraved Barrel Color |
Low magnification | Green |
Medium magnification | Blue |
Working Distance and Aperture Size
Objectives are also categorized by their working distance values.
- Ultra-long Working Distance: Enables imaging specimens where extra space is required.
- Shorter Working Distances: Provide greater resolution through wider light acceptance angles.
Infinity Optics and Field Sizes
Infinity optics support imaging across a wide field size with sharp resolution, beneficial for circuit inspection, biomedical microscopes, or light fluorescence tasks.
Specialized Objectives for Unique Applications
Some objectives cater to unique needs with specialized coatings and optical elements. Examples include:
- Phase Contrast Objectives: Enhance contrast in specimens with air or in biological applications lacking natural fluorite.
- Dark Field Objectives: Highlight specimens against a dark medium background.
- Reflective Objectives: Optimize performance across irregular surface layers in advanced microscopy settings.
Ensuring Compatibility and Best Practices
Referencing Manufacturers
Checking the degree of correction can help avoid image degradation. Consulting guides from reputable entities like the Royal Microscopical Society or authoritative researchers such as Michael W. Davidson from the National High Magnetic Field Laboratory can improve the interchangeability experience.
Matching Antireflection Coatings with Imaging Needs
Advanced thin-layer optical coatings mitigate unwanted reflections and light intensity fluctuations, allowing improved objectives to deliver high-contrast images. Combining these features ensures the optical microscopy setup supports dynamic needs.
What are infinity-corrected objectives, and are they compatible across microscopes?
Infinity-corrected objectives project light rays into an infinite focal plane, requiring a tube lens to create an intermediate image. Compatibility depends on the mechanical tube length, imaging medium, and specific design parameters, like light path alignment. Some microscopes adhere to international standards, improving the potential for interchangeability.
What factors affect the interchangeability of objectives?
Several factors affect whether objectives can be swapped:
Factor | Details |
---|---|
Thread Size | Determines fit with the microscope nosepiece; common sizes include metric 25-mm and 32-mm threads. |
Parfocal Distance | Refers to the distance from the objective mounting thread to the focal plane. Differences can misalign focus. |
Numerical Aperture (NA) | Influences the light-gathering ability and resolution; higher NA objectives may not align with all systems. |
Optical Tube Requirements | Systems may require infinity optics or specific tube lens designs for proper imaging. |
Coating Technology | Multilayer antireflection coatings or single-layer lens coatings impact light transmission and compatibility. |
How do manufacturers indicate compatibility of objectives?
Manufacturers use color-coded rings and engraved information to denote numerical aperture, magnification, and imaging media. For example:
- Black Color Code: Denotes specific numerical aperture settings.
- Blue Color Code: Indicates compatibility with certain magnification plans.
- Green Barrel Engravings: Highlight infinity optics.
Can objectives with specialized coatings be swapped between microscopes?
Objectives with advanced multilayer antireflection coatings or thin-layer optical antireflection coatings may not perform optimally across different systems. Optical elements designed for specific spectral ranges or wavelengths of incident light could result in chromatic aberration or image degradation when used incorrectly.
Why does an objective not focus properly?
Common causes of improper focus include:
Issue | Solution |
Incorrect Parfocal Distance | Adjust the focus or use objectives with matching parfocal distances. |
Thread Size Mismatch | Verify the nosepiece’s metric thread size (e.g., 25-mm or 32-mm). |
Light Path Misalignment | Check for alignment in the optical tube or microscope tube. |
Improper Immersion Medium | Use the correct liquid for immersion lenses, such as homogeneous immersion or dry lenses. |
Damaged Optical Surfaces | Inspect the objective barrel or external lens surfaces for scratches or dirt. |
What should I check if image quality deteriorates?
Reduced image quality can result from internal reflections, spherical aberration, or incorrect alignment. Verify:
- The light source image intensity and brightness of illumination.
- The alignment of the intermediate image plane and rear aperture.
- That the objective magnification matches the tube lens requirements.
How does the imaging medium affect performance?
Incorrect imaging media (e.g., using air instead of an immersion liquid) can cause dramatic improvement or deterioration in image brightness and quality. Refer to manufacturer guidelines for proper use.
How to choose an objective for specific applications?
Selecting an objective depends on several application-specific requirements:
Application | Recommended Objectives |
Biological Applications | Apochromat objectives or fluorite objectives for high-magnification imaging and color correction. |
Epi-Illumination | Plan achromat or infinity-corrected objectives for flat field imaging. |
Circuit Inspection | Long working distance or adjustable working distance objectives for hard-to-reach specimens. |
What role do advanced coatings play in objective performance?
Multilayer coatings, such as those using magnesium fluoride, enhance light transmission and reduce internal reflections. Single-layer coatings or quarter-wavelength antireflection layers are often suitable for standard achromats. Objectives featuring advancements in lens design and antireflection coating technologies achieve higher transmission values and minimize reflection losses.
Can interchangeable objectives improve performance?
Swapping objectives for high-performance objectives or those with apochromatic aberration correction can provide:
- Improvement in light-gathering power.
- High-contrast images across a broad range of visible wavelengths.
- Enhanced flatness of field and field curvature correction.
For example, using a 60x apochromat objective with a high numerical aperture can yield finer, diffraction-limited optical microscopy results.
Additional Insights
How are objectives maintained for optimal performance?
To maintain objectives:
- Clean external lens surfaces with approved solutions to avoid damaging specialized coatings.
- Store objectives properly to prevent damage to the objective barrel or internal lens elements.
- Ensure alignment of the microscope nosepiece and turret objectives.
References and Notes
For comprehensive insights, visit authoritative sources like the National High Magnetic Field Laboratory led by Michael W. Davidson at East Paul Dirac Dr. They provide extensive knowledge on advanced objectives, specialized coatings, and optical microscopy best practices.
Final Takeaways
Microscope objectives are conditionally interchangeable, requiring alignment of thread size, parfocal distance, numerical apertures, and tube lengths to maintain optimal performance. Matching objectives with the microscope tube type and application ensures reliable outcomes.
Consider improvements in antireflection coating, field curvature correction, and light transmission properties when selecting objectives. Whether for basic biological imaging or advanced high-magnification applications, an understanding of design parameters and specialized objective types will result in effective compatibility.